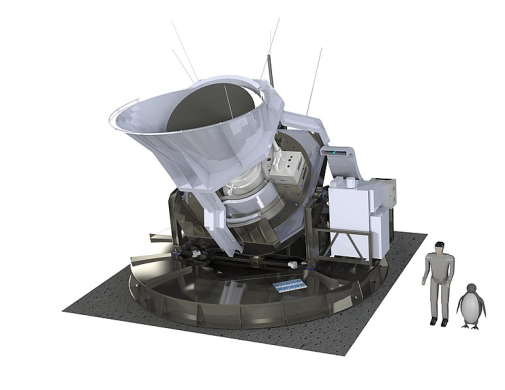
The Simons Observatory is an experiment designed to observe the microwave sky using 60,000 cryogenically cooled detectors. The experiment will set the stage for the next generation of experiments studying the cosmic microwave background.
At an altitude of 5200 m, the Chajnator plateau offers one of the best locations in the world for observations at millimetre wavelengths. This is where the Simons Observatory will field four telescopes currently under construction. Three small (0.5-m) aperture telescopes (SATs) are specifically designed to observe the polarized anisotropies on degree angular scales, while a large (6-m) aperture telescope (LAT) will focus on arcminute-scales. Renderings of the Small and Large Aperture Telescopes, with humans (and penguins) for scale, are shown here. Once deployed, these telescopes will represent one of the world’s most sensitive CMB experiments.
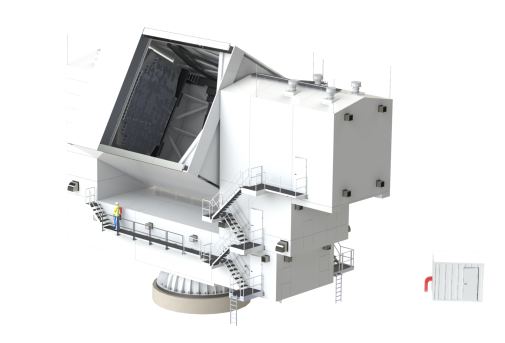
The Simons Observatory will tackle a range of questions in cosmology, but two key science goals are: 1) the search for primordial gravitational waves via mapping of swirly polarization patterns in the CMB polarization field often referred to as B-mode polarization; and 2) the mapping of the integrated lensing potential of the observable universe via the so-called lensing B-mode polarization.
Jon Gudmundsson, a senior research scientist at Stockholm University, just returned from Vertex Antennentechnik in Duisburg, Germany, where he assisted with the factory acceptance test of one of the telescope mounts for the small aperture telescopes.
The SATs, which sport a rather unique optical baffling design clearly visible in the video, will demonstrate a number of technological advancements, including: 1) a continuously rotating half-wave plate supported by a superconducting magnetic levitation bearing; and 2) three 42-cm diameter silicon lenses with metamaterial anti-reflection coatings specifically designed to minimize internal reflections across a wide band of operating frequencies.
These three concentric silicon lenses, which are cooled to 5-Kelvin, will focus radiation from the sky down to the detector elements. The detectors then measure variations in the sky temperature by coupling the incoming radiation to a thermally isolated dielectric chip that is cooled to 100 mK! This chip also includes a voltage-biased superconducting transition edge thermometer which functions as a ultra-sensitive thermometer.
Before making it to the detectors, however, the polarization of the incoming light is altered by the achromatic (wide-band) polarization modulator plate that rotates continuously in order to help separate the incoming signal from spurious signals that are generated within the telescope — a part of the systematic mitigation strategy.
Distributed between the four telescopes, the experiment will deploy detectors sensitive to six frequency bands ranging from 30-270 GHz. The broad frequency coverage helps the experiment distinguish signals caused by polarized emission in our own galaxy from any potential primordial signal.
Wide frequency coverage and great sensitivity are however not the only requirements for a successful experiment. The instrument design also needs to steer clear of various systematic effects that can produce false polarized signals in the maps. This aspect becomes more important as experiments push down constraints on primordial B-mode amplitudes. A particularly large class of potential systematic effects is related to optics.
Researchers at Stockholm University have been developing tools that speed up the optical design and characterisation process and allow one to quantify the impact of optical systematics on the search for primordial B-mode polarization. One aspect of this work involves developing a full sky beam convolution algorithm that can generate realistic time domain simulations. In a 2019 MNRAS publication, A. Duivenvoorden, a recently graduated PhD student at the Oskar Klein Centre and the Stockholm University Physics Department, showed how we can scan the sky with a mock satellite experiment to generate more than 1e12 detector samples from the sky while accounting for various optical imperfections. Together with Duivenvoorden (now at Princeton University), Stockholm University PhD students, Alexandre Adler and Nadia Dachlythra, as well as University of Bologna PhD student Matteo Billi have now been upgrading this framework so that it can incorporate optical effects from polarization modulators such as the ones to be deployed on Simons Observatory Small Aperture Telescopes.
In order to make full use of this time-domain simulation framework, we need advanced optical simulation capabilities. Part of that effort is described in a new Simons Observatory publication.
By combining time-domain beam convolution algorithms with state-of-the-art optical simulations, we will better understand how our telescopes conspire to create false (systematic) polarization patterns. The end result will hopefully lead to more accurate interpretation of our data and therefore our cosmological results.