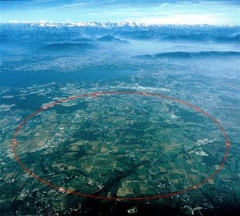
ATLAS is one of the four large detectors at CERNs Large Hadron Collider, LHC. Using this detector we adress a number of open questions when it comes to our understanding of the smallest building blocks of matter.
In the LHC protons are accelerated close to the speed of light in two rings where the protons move in opposite directions. At the interaction points where the protons collide there are gigantic detectors used to investigate the results of these interactions. ATLAS, the detector where the research group at Stockholm University is active, is the size of an eight-store building placed in a large cavern 100 m underground.
Our research group has contributed to the design and constructions of two sub-components of the detector, namely electronics for the triggering system and read-out electronics for one of the calorimeters in ATLAS.
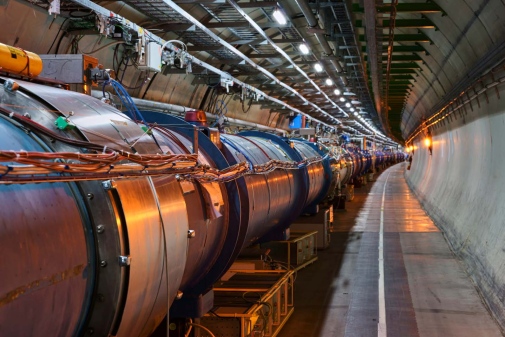
It is essential to have the ability to predict all elementary particle reactions, in order to construct models of the Big Bang, to understand the composition of the past, present and future Universe and extract the fundamental symmetries that rule over its evolution.
The Standard Model (SM) of particle physics provides an accurate description of electroweak and strong interactions up to several 100 GeV. However the Standard Model does not account for observed phenomena such as dark matter (DM), neutrino oscillations and non-zero neutrino mass, dark energy, or gravity. Many alternative theories for how to extend the SM to incorporate these Beyond the Standard Model (BSM) phenomena have been proposed.
The task of the ATLAS experiment at the LHC is to experimentally constrain and determine the correct high-energy and small-scale description of Nature using precise measurements of known particles, rare processes and seeking or excluding new phenomena and constraining or ruling out Beyond Standard Model theories.
The emphasis of the physics analyses of our group has been on trying to discover phenomena that cannot be explained within the current Standard Model of particle physics, that could help to resolve some outstanding issues in our understanding of nature, like what is the nature of the dark matter in the universe, and why is the mass of the newly discovered Higgs-boson so light when there are good theoretical arguments for it to be much heavier.
These studies follow two paths either by searching directly for production of new particles or by making precision studies of the heaviest of the known particles - the top-quark. Modifications to the standard model parameters or the existence of so far unknown particles could modify the properties and interactions of the top-quark in a detectable way. Our group is currently involved in:
- Precision tests of standard model physics, in particular processes where the top quark is produced.
- Searches for particles that could form the dark matter in the universe.
- Searches for exotic particles like leptoquarks and heavy long-lived particles.
Precision tests of standard model physics
We use precision tests of the standard model predictions as a tool to search for signs of "new physics" — phenomena not described by the standard model. Even if the particles associated with potential new physics phenomena, becoming manifest at high energy, are not actually produced, they can still leave significant footprints in events at low energy. A well known analogous example of this effect is nuclear beta decay. The beta decay is modelled by Fermi theory which is effectively a four-point interaction at low energy, but originating from the mediation of a particle at much higher energy scale, the W boson.
By measuring the parameters of the Standard Model (SM), where the SM is interpreted as an effective theory at low energy, one is able to constrain and potentially discover new physics at a slightly higher energy scale via quantum fluctuations. In the Stockholm group we have focused on the effects affecting the SM top and higgs sector, mainly because the origin of the higgs mechanism is not known and the top-higgs interactions tend to dominate the quantum fluctuations at high energy. Processes that we look at are top pairs in association with a boson and di-higgs final states. An example of a surprisingly rich process with strong high energy behavior is Wt scattering, schematic diagrams are shown in the figure below.
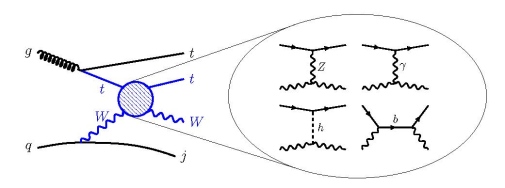
Searches for particles that could form the dark matter in the universe.
Dark Matter particles could be produced in proton-proton collisions at the LHC, either directly or in association with other particles or even in the decay of heavier particles.
The ATLAS experiment allows to probe large regions of parameter space (coupling strength with standard model particles, and dark matter particle mass) inaccessible to other direct and indirect Dark Matter searches. The Stockholm University group searches for Dark Matter with monojet, leptonic and heavy flavour signatures. Several models are investigated: Supersymmetry models, effective theories, simplified models and simplified plus scalar particles.
Searches for exotic particles like leptoquarks and heavy long-lived particles
There are good reasons to expect that the LHC will see new particles. However, we don’t know what the properties of those particles are. How heavy are they, what is their charge, and crucially, how long do they live ? At SU we are looking for massive long-lived particles. The particle lifetime is typically around 1 nanosecond (10-9 s) which, in everyday life, is rather short. However, most particles decay more than a million million times faster so the particles we look for are very special.
We search for particles produced in the proton-proton collision and which move a few centimeters at speeds near to light speed before decaying. They leave a signature of a displaced vertex — lots of particles emerging from the decay at a position distant from the proton-proton collision as can be seen in the figure. These particles can be measured by the ATLAS detector and the position of a vertex reconstructed.
Long-lived particles are predicted in a range of theories beyond the Standard Model such as supersymmetry and extra dimensions.
At Stockholm University we are looking for displaced vertices arising from the decay of massive particles produced in events with a range of other objects such jets, muons, and missing transverse energy.
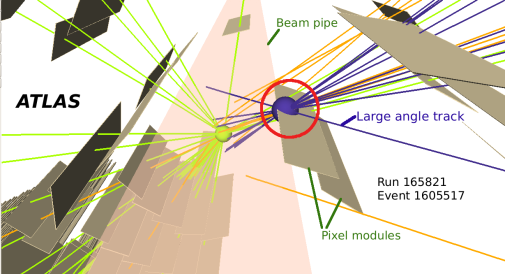